Micro fuel cells have been a promising technology for years. Are they
ready to start delivering on their promise?
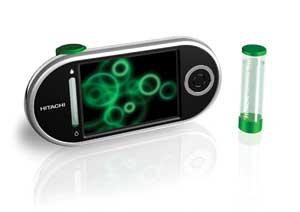
Click here to enlarge image
|
Micro fuel cells offer a potential replacement for batteries in
portable applications. Invented in 1839, fuel cells first gained public
attention when NASA used them in the 1960s for the Apollo space missions.
Fuel cells have been powering submarines since the 1980s and buses and
cars since the 1990s. The question is whether their little brothers, micro
fuel cells, should be taken seriously in portable applications any time
soon.
First off, a fuel cell is not a battery. A battery is an energy storage
device that provides power as needed. It “starts up” instantly, needs
lengthy recharging, and wears out after 300-500 charge cycles. A fuel
cell, in contrast, is a power generator that provides constant power,
needs time to warm up, can be recharged quickly, and wears out after
several thousand hours.
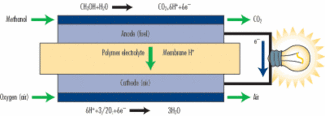
Figure 1. Operation of a direct-methanol fuel cell.
(Figure courtesy of Toshiba) |
A fuel cell burns hydrogen in the presence of oxygen, produces
electricity, and generates water, CO2, and heat as byproducts. There are
three main types of fuel cells:
•Direct hydrogen, which obtains hydrogen fuel from compressed gas,
metal hydride, or chemical hydride
•Fuel processing, which externally processes hydrocarbon fuels that are
rich in hydrogen, such as methanol (reformed methanol fuel cell, or RMFC)
to generate hydrogen on demand, which is then fed into the cell
•Direct conversion, which directly uses liquid hydrocarbons, such as
concentrated or neat methanol (direct methanol fuel cell, or DMFC), from
which the hydrogen is separated in the cell itself.
All fuel cells use the same basic structure: an anode and a cathode
separated by a liquid or solid electrolyte. A catalyst, such as platinum,
is often used to speed up the reaction at the electrodes.
There are two major system architectures for portable fuel cell
systems: active and passive. Active systems have pumps to push air onto
the cathode; condensers to separate out the water vapor that comes off the
cathode; tanks to mix it with incoming methanol to maintain the proper
mixture; and pumps that move the resulting fuel to the anode. Passive
systems eliminate all of these components and rely on fuel injected
directly onto the anode and ambient air circulating to the cathode.
Passive systems are less complex and expensive than active ones, but they
generate less power and are less efficient.
The type of fuel cell most suited to small portable applications is the
DMFC. These cells (Figure 1) can directly use liquid methane at the anode,
where the methanol is first split into hydrogen and carbon dioxide before
a special platinum/ruthenium catalyst splits the hydrogen into protons and
electrons. The protons then diffuse across the polymer membrane to the
cathode, while the electrons pass as current through the external circuit.
At the cathode the electrons recombine with the protons and with oxygen to
form water, which, along with the carbon dioxide, is then passed out of
the system. The voltage generated by a single DMFC cell is 0.3-0.9 V,
though cells are typically arranged in stacks (Photo 1) of varying sizes,
depending on the application requirements.
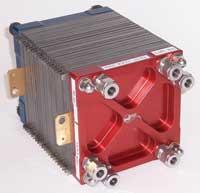
Photo 1. A fuel cell stack. |
DMFCs have a potential advantage over LiIon batteries that has proven
elusive: higher energy density. While pure methanol has a 10X greater
energy density than LiIon by volume and 30X by weight, in practice this
advantage remains theoretical. DMFCs typically operate at about 30%
efficiency, wasting as much as 70% of the energy produced as heat. Much of
the problem is due to the tendency of methanol to cross over the membrane
into the cell instead of reacting at the anode.
To counteract this problem, many manufacturers dilute the methanol, but
this in turn reduces the energy density of the cell. This can result in
larger cells to achieve adequate power output, further diminishing the
attractiveness of fuel cells versus batteries.
To compare energy densities in a meaningful way, you need to plot
weight against runtime. If the LiIon battery pack in your notebook
computer will power it for four hours, how much will the fuel cell and the
required fuel weigh that can power it for the same duration-and how large
will they be? With current technologies, your notebook battery will show a
higher energy density than a fuel cell. But if you increase the
timeframe-forcing you to add more and more batteries to complete the
“mission”-the fuel cell’s density quickly passes that of the batteries,
since the weight of the additional fuel is far less than the weight of
additional batteries. This may seem an odd scenario when discussing a
laptop computer, but it is quite meaningful if you’re a foot soldier
carrying a large load of batteries for an extended period-a key target
market for fuel-cell manufacturers.
Fuel-cell makers take different approaches to dealing with the
limitations of DMFCs. Using a patented circulation system, MTI Micro Fuel
Cells’ Mobion DMFC runs on pure methanol, eliminating water as an
emission. MTI claims that its Mobion test units, customized to the same
size and shape as the U.S. Army’s standard BA5590 portable battery, ran
continuously on one integrated fuel tank to achieve twice the energy
output.
Neah Power Systems makes a silicon-based DMFC, replacing the 10-mil
polymer membrane with a 400-micron silicon honeycomb containing a
catalyst, giving a much larger reactive area. Neah uses an enclosed,
all-liquid system, using methanol as the fuel and nitric acid as the
oxidizer. With an eye toward the military market, Neah claims its cells
can decrease the weight soldiers have to carry related to portable power
by up to 65% over a 72-hour mission.
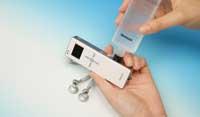
Photo 2. Toshiba’s DMFC-enabled MP3 player.
|
UltraCell Corp.’s UltraCell25 attaches hot-swappable cartridges to a
DMFC that runs on 65 % methanol. The UltraCell device places a reformer
stage that takes in methanol and outputs hydrogen to the cell stack.
Ultracell claims that its cell with two refill cartridges achieves an
energy density of 340 Wh/kg versus LiIon’s 160 Wh/kg, yielding a 50%
weight saving (3.5 lb versus 7.3 lb) when running a 20-W laptop for 24
hours.
Not surprisingly, manufacturers of portable electronic devices are
looking seriously at micro fuel cells. Motorola, Toshiba, NEC, Fujitsu,
Hitachi, Samsung, Sanyo, and LG, among others, all have active fuel cell
programs, have demonstrated prototypes, and are considering the commercial
viability of their products. At this point Toshiba has gone farther than
most and recently demonstrated a DMFC-powered portable audio player (Photo
2) that runs for four hours on a charge, at which point you recharge it
with a squirt of pure methanol from a pocket dispenser. The company has
also demonstrated a DMFC-powered cell phone, which it is developing with
Japanese carrier KDDI. The phone weighs 150 gm, of which the fuel cell
contributes 40 gm. Toshiba claims the cell generates 7 Wh, enabling 2.5X
longer talk time than would be possible with a LiIon battery.
While technically viable, micro fuel cells won’t start stealing major
market share from LiIon batteries in cell phones, PDAs, and notebook
computers anytime soon. Part of the reason is the nature of fuel cells:
they’re power generators, not batteries. They put out constant power, and
most portable devices have variable power requirements. If your fuel cell
is putting out 20 W when your notebook only needs 5 W, you’re wasting
power.
It’s possible to meter the fuel just as a car meters gas to the engine,
and manufacturers are doing that using the SMBus in notebooks. But it’s
not yet possible to respond to power surges as quickly as a battery, so
you’d need to have the fuel cell put out more power than you need at any
given time-unless you use the fuel cell in combination with a battery,
which may make the most sense in many applications, such as recharging
your notebook battery on a flight from New York to Beijing.
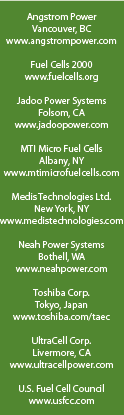
|
DMFCs produce water vapor as a byproduct-not something you want to
introduce in an electronic device. They can also generate a fair amount of
heat, another no-no. Active or reformer-type cells, while generating
higher power than passive DMFCs, have noisy pumps and fans. DMFCs use
exotic metals (usually platinum) as a catalyst, making them relatively
expensive. And durability remains an issue in both active and passive
cells. Finally, while the fuel-cell engine may be comparable in size to a
battery, the fuel adds bulk, weight, and cost. Now that rechargeable
batteries have all but replaced disposable ones in portable devices, will
consumers be willing to pay to run their devices?
There are regulatory issues, too. No U.S. or European airline will
allow passengers to carry methanol cartridges onboard. In October 1995 the
U.S. Fuel Cell Council offered proposed standards to the International
Civil Aviation Organization (ICAO), which they hope to see approved in
2006.
Many of the objections raised here have been addressed in products that
are already sampling. You can probably expect to see DMFC fuel-cell
battery chargers as well as replacements for notebook batteries in time
for next Christmas. But serious market share will have to wait for further
refinements in micro fuel cell technology, not to mention price.
PD
Portable Design January, 2006
Author(s) : John Donovan
|