Betting on Batteries (Part2)
by Joshua Israelsohn
Contributing Technical Editor
February 15, 2008
The last installment of The Casual Observer examined batteries–the
oft-maligned portable energy source on which many of our modern electronic
devices depend. I observed then that, kilogram for kilogram, modern cells
are the most space- and mass-efficient portable sources of electrical energy
to which we have ready access. One possible cause for their poor reputation
is that we tend to think of them as voltage sources–a role they play rather
poorly. But as energy sources with unregulated voltage outputs they are more
attractive with energy densities ranging from about 144 to nearly 600 kJ/kg.
Unfortunately, a battery's capacity is nonlinearly parametric in output
current, temperature, and charge-cycle count. This behavior, even over a
conservative range of operating conditions, can make estimating their
remaining charge difficult, which is where battery fuel gauge ICs come in.
Fuel gauge ICs have been around for many years, but trends in a few
applications have pushed some of them to a level of sophistication not in
evidence in their earlier generations.
At present, there are two common approaches to fuel gauging: The oldest is
the coulomb counter. This device integrates current to track the charge into
and out of the battery:
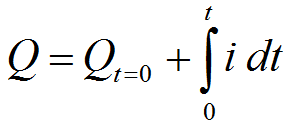
This approach would work fine by itself if batteries were made of ideal
cells. They are not, however, and straight coulomb counters, as a result,
offered poor to marginal accuracy. Modern day coulomb counters, however,
improve significantly on the basic design by including various combinations
of temperature, load-current, and cell-aging compensation to better model
cell behaviors. The second approach measures the battery's output impedance
and compares the measurement with characterization data developed in
cooperation with the cell manufacturer. Examples of both methods include
zero-current (or near-zero-current) voltage measurements to tighten the
correlation between battery models and actual battery behavior.
Battery fuel-gauge ICs currently available claim accuracies from about 1 to
6% depending on model. The real attainable accuracy is difficult to assess,
in all candor, because most devices' spec tables report only the accuracy of
their various measurement capabilities but not the conformance of the
remaining-charge prediction to the pending experience. Although there is not
a strict correlation the general trend is that greater claims of accuracy
accompany devices that require more complex or proscribed design-in
procedures. These include larger software loads and more narrowly defined
cell sources to minimize the spreads in cell-model parameters.
At the OEM level, the current use of battery fuel-gauges appears to
significantly lag current ICs' capabilities. With the exception of
laptop-computer batteries, which include gauges within the cell packs and
reporting software available on the desktop, few portable devices offer
charge-state information to the user at a resolution commensurate with gauge
ICs' claimed accuracy. Remarkably, this is true despite evidence that
suggests that the devices themselves depend on greater resolution for
internal monitoring and control functions than they report to the user:
Like laptop computers, PDAs, wireless handsets, and other portable devices
that provide data-intensive functions require an orderly shutdown to prevent
data loss. For these devices, the charge-state data that battery gauges
provide help the system supervisor initiate a shutdown process while
sufficient charge remains to ensure no data loss. Depending on the device
and the size of its battery, the shutdown may initiate with as much as 5 to
10% reserve capacity. Good gauging accuracy allows users greater run time
per charge cycle by providing more reliable charge-reserve estimates.
Whether they cost $30 or $300, wireless-handset displays, however, rarely
show the user more than a four-segment indicator–a resolution of 25% with
none of the interpolation possible with analog indicators like your
automobile's fuel gauge. So, though the system may track the battery charge
to something in the neighborhood of five times the displayed resolution,
users don't get the benefit of those measurements to guide their usage
decisions. Rather than risk an ill-timed end of a discharge cycle, many
users tend to recharge their portable devices early in the cycle's second
half. This behavior can reduce the user's perception of per-charge runtime
and, as a result, needlessly reduce customer satisfaction.
Systems equipped with battery gauges usually present charge-state
information to users in one of two modes. Most commonly seen on laptop
computers, one mode presents the charge state in terms of remaining runtime.
Wireless handsets, digital cameras, and other portable devices usually
preset charge-state information in terms of the fraction of full charge
remaining. Of the two, my experience is that the former is essentially
useless and the latter is quite helpful, particularly if the display
resolution is, say, in increments of 10% or smaller.
The problem with interpreting the battery's charge reserve in terms of
remaining runtime is that it forces a calculation based on operating
conditions that will not hold steady for the duration of the discharge
cycle. Indeed, if one thing can be said about this kind of display it is
that it is guaranteed to be wrong. The error is greatest at full charge and
tends to get better as the system approaches its end-of-charge point. Of
course, at the end-of-charge point the estimate is both correct and
irrelevant. This behavior parallels that of an automotive GPS system's ETA
(estimated time of arrival) display. At the beginning of a journey the ETA
error is large because the system bases its calculation on driving-speed
assumptions that do not necessarily reflect the prevailing driving
conditions. The estimate continuously improves as the progress along more of
the path becomes a matter of experience and less of it remains in the realm
of prediction until you reach your destination at which point the
calculation becomes both perfect and perfectly useless.
Expressions of charge state in fractional terms of a full charge are less
ambiguous and more useful to users. This display parallels your car's fuel
gauge, which reports the amount of fuel in the tank, not the distance you
might yet travel on the reserve.
Not all portable devices benefit equally from fuel gauging and not all
gauging techniques come with equal design-in and BOM costs. Examine the
value that gauging brings to your product and consider the incremental value
of accuracy within the contexts of both the system's and operator's uses for
the information.
For this sort of exercise, I tier portable applications into four levels.
The top tier comprises battery-powered devices upon which human life or
safety may depend. This category might include dramatic examples such as
portable medical equipment but I'd also include less exotic systems such as
GPS navigational devices.
Devices that depend on an orderly shutdown at the end of a discharge cycle
populate my second tier. These devices often operate with file systems that
can sustain damage if the system's energy supply fails unexpectedly. This
category includes devices such as laptop computers, PDAs, and multifunction
wireless handsets.
The third tier comprises devices that capture data. This group includes
common consumer electronic devices such as digital cameras, video recorders,
and audio recorders. It also includes battery-powered measurement subsystems
such as remote utility meters and industrial monitoring equipment.
The last tier includes devices that present or display information already
captured in some stable storage medium. These include portable media players
and other devices the interruption of which are least likely to cause loss
or harm.
Like many perspectives, this one has limits on its applicability. For
example, though smoke detectors and carbon-monoxide alarms clearly fit into
my top tier, a simple and conservative threshold detection circuit might
better serve the end-user than the most accurate battery gauge: In
applications like these, keeping the final product costs down improves the
likelihood that consumers will deploy and periodically replace the devices.
Improving the market penetration of such safety devices is certainly worth
sacrificing even a large fraction of a battery's charge cycle–a tradeoff
that exemplifies how each application suggests its own valuation of battery
fuel-gauging ICs.
|