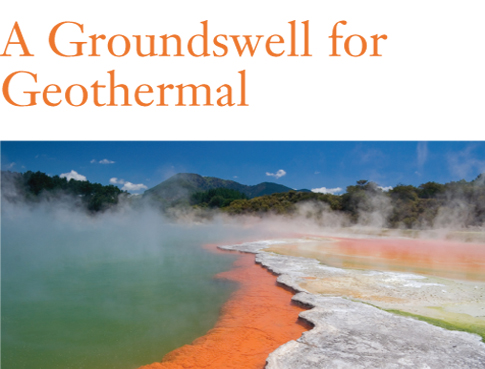
Though the US is the world’s largest producer of geothermal electricity,
generating an average of 16-billion kWh of energy per year, this is still
only a fraction of what it could be producing.
By Peter Hildebrandt
When we think geothermal energy, many of us might consider Yellowstone
National Park, Hot Springs, Saratoga Springs, Iceland, or other “hot spots”
scattered around the world, as chief sources of such energy. But geothermal
energy means much more. It is now recognized as an energy source capable of
supplying at least 20% of the energy needs of the US, according to the
Geothermal Energy Association (GEA). The ultimate source of all this energy
is the decay of radioactive potassium, thorium, and uranium at the earth’s
core. This energy, in turn, heats up the earth’s mantle and crust. Resulting
large blocks of molten material constantly recycle within the earth.
Fortunately for us, the radioactivity is also blocked from harming us by
that same mantle and crust.
The National Renewable Energy Lab estimates that domestic resources of
geothermal energy are equivalent to a 30,000-year supply of energy at our
current rate of use in the US. If just a small percentage of the vast
supplies of this resource are recovered, it will make a large impact on our
nation’s energy supplies. Estimated accessible geothermal resources for the
US amount to a staggering 10- to 67-billion barrels of oil equivalent, and
estimated developable geothermal resources come to 4.2 to 9.5 barrels of oil
equivalent. Armed with these statistics, one cannot help wondering why more
energy operations of all sizes aren’t jumping on the bandwagon. One simple
reason—among a few others—might be simply availability of the proper-sized
equipment for small- to mid-sized developments.
Geothermal electricity, ground-source heat pumps (GSHPs), and direct use (a
method documented as early as ancient Roman times) are among the many varied
ways of using geothermal energy. These methods use the resource directly to
varying degrees. GSHPs, for instance, simply take advantage of the
comparatively constant temperature of the earth below the surface, using it
as a source and sink for both heating and cooling. For cooling, heat is
extracted from the space being dealt with and dissipated into the earth,
whereas for heating, heat is taken from the ground and pumped into that same
space.
Geothermal Electricity
An example of a small commercial operation is the 400-kW Chena Hot Springs
Geothermal Project in Alaska (the state’s first geothermal power plant),
which would fit in many other places around the US, according to Karl Gawell,
executive director with the Geothermal Energy Association, a geothermal
energy trade group. “The situation with the Chena Hot Springs Geothermal
Project, and the size of this development, reminds me of what’s happening in
the wind power industry,” Gawell adds.
“If people had to build their own small wind turbines, most people would not
be using wind power, because it would be too hard to build one yourself,” he
says. “But, today, there are small wind developers out there, who are
looking at the distributed wind generation, and they are providing the
product. This is one of the keys to getting distributed generation going.
People must be provided with the equipment. With the Chena Development, the
thing that has everyone excited is that it’s a small power unit that came
out in 2006, and one that United Technology Corp. is talking about mass
producing. So, one, there is an equipment provider, and two, this is a real
example out there that people are learning from. Once the learning curve is
established, and as things move forward, there’s a need for someone really
interested in providing small power equipment, as well as builders willing
and able to construct a few of these sites, so that there’s real-world
learning.”
The use of these small units is even coming into play in oil and gas fields.
Gawell feels that if there is a market in turn, opening up for such small
power systems and their equipment, the units will be even more available.
“In west Texas, for example, as investigated and studied by Southern
Methodist University [SMU], many oil and gas wells, as they age and get
deeper, are also bringing up lot and lots of hot water,” Gawell says. “In
certain settings, for every gallon of oil recovered from a well, 9 gallons
of hot water surface as well. The question then became: Why couldn’t the hot
water itself be used as a source of energy to run the power system and pay
for all the pumping, instead of having energy used, both in its extraction
during well operations and in its re-injection into the ground?
“In fact,” he continues, “there is no reason why excess power from this
source could not be sold back to the grid. The very first demonstration of
such an operation is taking place in Wyoming at the Rocky Mountain Oil and
Gas Testing Center, 35 miles north of Casper. A small power system, built by
Ormat Technologies Inc., will be used to run the pumps for the
fields—they’re essentially going to self-generate to run themselves.”
According to Gawell, SMU has concluded that there are hundreds of such sites
where this type of small power unit would be exactly the right type of
equipment. It would be best if new infrastructure did not have to be built
and the unit could simply be transported on the back of a truck to the
oilfield site and use the hot water to run the system, as the power lines
are already in place. “Companies are seeing that there may be a market for
small power systems at such sites,” Gawell adds.
As a trade association, six to seven years ago, the GEA changed its goal
from stressing to companies the benefits of geothermal energy to emphasizing
the expansion of the use of the geothermal resource itself. It works a great
deal with public interest groups, environmental groups, and others. “If
that’s our primary goal everybody gains,” Gawell says. “We can’t be worried
about whether or not every company out there is going to win. We’re trying
to grow the whole market and expand how we use this inexhaustible resource.
If your company has any smarts, you’ll figure out how, if the market’s
growing, you can do well. As with other renewable resources out there, they
all are enormous resource bases. It’s just a question of learning how to use
them, making the commitment to say we want to do it. It’s not just utility
power; it’s also distributed generation, home-heating and other things. We
need to learn how to use this resource to its fullest in accomplishing these
objectives. The potential has always been there; that’s never been the
problem.”
Ground Source Heat Pumps
The International Ground Source Heat Pump Association (IGSHPA) has done
training, conferencing, and many research programs over the past 26 years.
It has watched the numbers grow into well over a million such systems
installed. Jim Bose, executive director of the IGSHPA, says geothermal or
ground-source heat pumps are popular for government buildings, many schools
and military housing. Much depends upon the company doing the work in this
area and how it sees its market, according to Bose. Some companies are
looking at residential uses, while other are looking to manufacturing. This
depends on the company’s size and who it can team with.
“A large company will have a subsidiary group that finances large projects,
and they can come in and bid on such projects as large military housing,”
Bose says. “They are able to bond it and do the work. It depends on the size
of the heat pump manufacturer and other kinds of allied businesses involved.
The earth makes for a more moderate environment than the air when it comes
to heat pump usage. It’s warmer in the winter and cooler in the summer than
the ambient air during peak conditions—that’s a real, big advantage. For
instance, if you had three wind turbines generating for your operation, if
you were then to hook up to a GSHP, you’d perhaps only need two turbines.
“But, I think what’s wrong with a lot of other renewable technology out
there is that the growth rates can be a bit halting and very much tied to
government subsidies,” he says. “With the GSHP technology, the rates of
growth have been reported to be around 35–40% over the last three years.”
(This is based on pipe, thermal grout, and thermal borehole testing sales.)
“This technology is doing that on its own without government subsidies or
even much PR.”
Bose sees a big part of the problem with any new technology being the fact
that change is something that’s hard for people to consider. “Someone may
learn about GSHPs, go to their HVAC person who might then say, ‘Well, that’s
just not reliable technology,’ et cetera, based on hearsay,” he says. “But,
with this technology, I guarantee it will work. Much comes down to
tradition; change is the worst thing out there for some folks. Another
stumbling block is that developers do not like incremental costs. They
prefer to do the construction and turn around and sell it. Builders don’t
see a GSHP as something they’re going to get their money back on. It’s hard
to ask for more money for a home, when the payback with saved energy is in
the future.
“With school boards, the military, or the government, such long-term
occupants are willing to buy off on this, as they see and care about saving
in the long-run,” he adds. “A tract home builder’s going to sell a house
based on the huge chandelier in the entryway. Sellers tend to buy all the
house they can get for the money. We need to do a better job of teaching
people how to invest and view the home purchase as an energy investment.”
But, Bose points out, there’s a lot of activity in the GSHP area in
Oklahoma, due to the fact that they’ve been working on this longer than most
areas of the country. The IGSHPA has trained some 10,000 installers,
engineers, and mechanical contractors now working around the country through
its training program, featuring trainers also located throughout the US.
Generally, the trainers are already in the business, making their living
installing GSHP systems and training on the side. The IGSHPA also trains
installers in China, Korea, and other countries. “The first and oldest
ground-source heat pump installed in this area is now 26 years old,” Bose
adds. “It’s the same old heat pump, same old ground heat exchanger—it just
keeps on running. We have no idea how long it’s going to last.”
Manufacturers Adapt to Needs
Dee Brower, senior product manager with ClimateMaster, sees GSHP systems as
still a fairly small segment of the heating and cooling market, though it is
growing much faster than the traditional systems. ClimateMaster manufactures
water-source heat pumps. The heat exchange medium is water, or in some cases
an antifreeze mixture, and the source of heat can be geothermal that’s
buried in the ground in a ground-loop, which the water circulates through
before circulating through the unit.
“There are still a lot of projects out there that use a water-source heat
pump, but they don’t necessarily use a ground loop,” Brower says. “They may
have a boiler to keep the water temperature above a certain temperature and
then use a cooling tower or evaporative-type of cooler to bring the
temperature down. It’s basically the same equipment, but it can be a bit
confusing because of the different methods.”
Geothermal, in this application, tends to refer more to a system that has
pipe buried in the ground, taking advantage of the natural heat exchange of
the earth. With over 50 years in the industry, ClimateMaster now
manufactures water-source heat pumps exclusively. It has a wide range of
products for single-family homes and has thousands of units in commercial
buildings, schools, and government buildings. Its work is 80% commercial and
20% residential. The types of ground loops used for GSHP systems are either
vertical or horizontal. The best one to use is site-dependent. Schools often
choose the horizontal loop, because they will place it under the football
field or parking lot and it tends to be less money to trench than to drill.
For an office setting, things are usually more restrictive. They have heavy
air-conditioning demands, and, consequently, need a lot of loops, so
vertical loops will almost always be used.
“One arrangement doesn’t necessarily work better than another,” Brower says.
“It’s simply a function of heat transfer as far as how much pipe you have in
the ground versus how much you need. Regionally, the Midwest tends to have
horizontal loops whereas the South tends to have vertical loops, due to
space restrictions. Much also depends upon how much land is available and
what the drilling prices are.”
Vertical tends to be a neater option. Not as much landscaping is required
after the completion of work. With vertical loops, the standard installation
typically requires one hole per ton of air conditioning. Each hole needs to
be about 4 inches in diameter and about 200–250 feet deep (this varies
depending upon the region of the country). Two pipes are inserted with a
U-bit at the bottom. At the top of each of the holes, the pipe is headered
into a larger diameter pipe.
A 2,000-square-foot house, with 4 tons of equipment, would have four 200–
250-foot boreholes, manifolded together with the supply and return piping
coming into the house connected to the heat pump. The heat pump can be
situated wherever it needs to be, depending on where the structure’s
ductwork is located.
The upfront cost has always been a market barrier, according to Brower.
Their systems are typically two to three times the cost of a conventional
one. “Most of the exposure right now is on the residential application side,
as heating and cooling bills grab people’s attention,” Brower adds. “It’s
not quite as eye-catching for companies as it would be for an individual at
the moment. The growth in the industry works out to around 20 to 25 percent
per year; if it grows much more than that you don’t really have the
infrastructure in place to sustain it. That infrastructure includes
installers and drillers for the ground loop.”
ClimateMaster was involved with a local “zero-energy” home using
photovoltaic on the roof, spinning the electric meter backwards as
electricity is generated and forward as it uses it. Ground-source heat pumps
have been used to offset the cost of the solar panels by enabling smaller
panels to be used. Solar and geothermal together form a highly complementary
cost and energy-saving method. “If you’re going to spend the money on solar,
you're probably better off to spend some of what you would have spent for
solar, on geothermal and, therefore, lower the total energy cost. There is
virtually no area of the country where geothermal cannot be used.”
Other local Oklahoma City projects involving geothermal include all the
area’s Habitat for Humanity Homes. Such a use as the Habitat Homes features
the best of both worlds, as these are for low-income people, and also have
the lowest utility bills, according to Brower. At a combined middle school
and high school just down the street from the ClimateMaster offices, the
middle school section has heat pumps which use geothermal. The high school
has conventional equipment. Though they are both about the same in square
footage, the middle school has half the operating costs. “Those are the
types of success stories you hear about when you talk about geothermal. It’s
always about operating costs,” Brower says. “But, when you look into it
deeper, because there’s no outdoor equipment, it’s also lower maintenance
and for schools, there is no problem with vandalism or theft of copper, and
aluminum from the units for scrap.”
Though problems in the housing market have had a lessening effect on
geothermal development for residential applications, Brower says that with
commercial applications, especially schools, where taxpayer dollars are
involved, growth steadily continues. “To my knowledge, every new school in
Kentucky is now geothermal.”
The new Colin L. Powell Middle School, in Matteson, IL, is 160,000 square
feet, and features 82 ClimateMaster water-source heat-pump units. The
project uses a nearby pond as the source of this GSHP system’s
water-to-water heat-exchange capability. A groundwater-well pump was also
installed in case of a potential drought, as the pond must be maintained at
a depth of 14 feet. The annual savings to the school are expected to be
around $70,000 or some 60% of a conventional HVAC system.
The higher initial cost for the system was largely offset by a grant,
awarded for energy-saving technology, from an Illinois electric-cooperative
association. The project involves total capacity of 280 tons (985 kW) of
water-to-air, and 180 tons (633 kW) of water-to-water heat pumps, with
outside-air-energy-recovery air handlers adding to the efficiency of the
overall design. “One of the biggest points in favor of using this type of
system is the fact that in each classroom, the teacher controls the
climate,” says Jerry Gillette, project manager with Globetrotters
Engineering Corp. in Chicago, IL. “Not only is there a thermostat in each
room, but the zoning is such that there’s a dedicated heat pump that does
both heating and cooling for each individual classroom.
“We also gain a maintenance advantage from the standpoint of isolation of
zones,” he says. “With a traditional variable air volume system, for
example, one unit will often handle more than one room—making it more
complex to deal with conflicting requirements in the different rooms than we
have with this water-source heat-pump system.”
Community and School with Long History of Direct-Use Geothermal
The Geo-Heat Center in Klamath Falls, OR, part of the campus of the Oregon
Institute of Technology (OIT), received the “Chill Out” award in June 2007
from the National Wildlife Federation, for its use of geothermal heat energy
on the campus. But, that is only the latest acknowledgment of an especially
innovative school when it comes to geothermal energy. The campus itself was
placed in its current location back in the 1960s, because of the geothermal
resources onsite. Geothermal heat energy was used in Klamath Falls by the
Native Americans for some 10,000 years.
The town itself has about 600 geothermal wells—mostly for private homes—and
the campus is heated entirely from geothermal energy derived from three
wells. This is 192°F degree water used to heat over 750,000 square feet of
space on the OIT campus. This saves the institution approximately one
million dollars in energy costs annually. It’s been heating the campus and
has all its space heating and domestic water heated through geothermal
energy. The District Heating System in downtown Klamath Falls heats some 20
buildings in addition to removing sidewalk snow through heat. Other uses in
the community include a local brewery using geothermal energy used in the
production of beer.
Based on geochemistry, those at the Geo-Heat Center believe 300°F water
exists at depth, so plans are in the works to drill a 6,000-foot-deep well,
and generate electric power. Calculations have shown that at least a
megawatt of power will be derived from this source, according to Geo-Heat
Center director and co-founder John Lund. This is enough to supply all of
the electricity for the campus.
“We’d be the first campus in the world 100% green when it comes to energy
use,” Lund adds. “Though there are some 800,000 geothermal heat pump units
in the country—some 50-60,000 units per year are being installed all across
the country—that’s not something we’re directly involved with on campus, but
we do provide technical assistance for geothermal heat pumps projects all
over the country. We are most involved with power generation and direct use
geothermal applications. Our Web site does, however, have pointers for
homeowners looking into using GSHP installations.”
Lund and the Geo-Heat Center are most excited about the contents of a new
report, recently released by MIT, on engineered geothermal systems, what is
sometimes referred to as “hot-dry rock.” “We’re excited by the conclusion of
this study, which states that if drilling is done to a depth of from two to
three miles—virtually anywhere in the US—enough energy will be yielded to
generate 100,000 megawatts of electricity by 2050, the year such energy
could be online from this source,” Lund says.
“Typically, the earth heats up at about two degrees Fahrenheit per each one
hundred feet of depth,” he continues. “Water would have to be pumped down a
well, be hydro-fractured, and a second well would have to be drilled to
intercept this water as it flows through the rock, picking up heat, and then
recirculating it in a system like a closed loop.
“The challenge would come in finding the places on the earth where this
would be the best most productive resource. Geophysicists could also direct
us in avoiding areas of potential hazard due to seismic activity.”
Peter Hildebrandt writes on engineering and scientific subjects.
© FORESTER COMMUNICATIONS, INC
P.O. Box 3100 • Santa Barbara, CA 93130 • 805-682-1300
To subscribe or visit go to:
http://distributedenergy.com |