The Limits to Renewable Energy
5.16.08 |
|
Don
Kopecky, Senior Supervising Engineer, WorleyParsons |
Part I of this article discussed the types of energy created from
various sources, and examined wind and bioliquids. Part II of this article
will continue with a discussion of energy from biomass, solar, and ocean and
river currents.
Biomass
The cellulosic materials which comprise biomass are many and varied,
including wood and woody plants, grasses, corn stover, sugar cane bagasse,
construction debris, municipal solid waste and yard waste. The potential
supply of cellulosic material is enormous; the U.S. Department of
Agriculture has estimated that as much as 26 quads (quadrillion, or 1E15
BTUs) could be available from biomass in the U.S. The difficulty lies in the
harvesting and transportation. Because the supply is scattered all over the
country, the transportation and handling costs can easily exceed their
value. However, the consumption of energy is also scattered all over the
country, so the challenge is to keep the consumers of biomass very near the
supply. While there are plenty of ideas for using biomass to manufacture
liquid fuels or generate electricity, its most practical application is in
stationary heating. Future conditions may change this situation, but we will
not consider that here.
Using biomass to heat dwellings is as old as mankind itself; it is only
during the last few hundred years that we have switched to types of fuel
which are considered to be more convenient. It still provides a large source
of our heating fuel, in 2006 approximately 2.8 quadrillion BTU of biomass
was burned in the U.S., compared to 0.7 quadrillion BTU of oil and 8
quadrillion BTU of natural gas. Currently, about 5.5 billion gallons/year of
oil are consumed for residential heating and this can be displaced by 40
million tons/year of biomass, or less than 3% of the available supply.
Presently, the major objections to burning biomass for residential heating
are the inconvenience, and the generally high emissions of air pollutants.
Modern technology has provided solutions to both these problems.
Pellet stoves are becoming enormously popular right now because they are
clean burning and much more convenient than chunk-wood stoves. Wood pellets
are very expensive compared to the value of their raw materials, about
$150/ton for pellets vs. $20/ton for hardwood pulp. Even at that price,
pellet heating is more affordable than fuel oil or propane ($11/mmBTU vs
$25/mmBTU) and comparable to natural gas ($12 mmBTU) so it is reasonable to
expect their use to grow. Currently there are an estimated 800,000 pellet
stoves in use in North America consuming 1.1 million tons/year of pellets.
Pellet consumption for heating is currently growing at a rate of about 15%
and there is no reason to expect that growth to stop. This currently
displaces about 0.02 quads of fossil fuel consumption compared to 4.6 quads
of fossil fuels still used for residential heating. Thus there is still
potential for this use of biomass to grow by a few orders of magnitude.
Burning biomass in blends with coal for the production of electricity is
also becoming more popular. Large coal fueled boilers are perfectly capable
of handling the ash contained in biomass, and are always equipped with
automatic combustion controls which optimize conditions so the fuel is
burned cleanly and efficiently. However, the boilers were built to burn
coal, and all their material handling systems were sized for using coal, so
there are some limitations to the fraction of biomass which can be blended.
The more important limitation though is the quantity of biomass which can be
economically supplied to a central location. This limitation fixes the upper
limit on the biomass which can be supplied to a single location at about
250MMBTU/Hr. While this is very small compared to the fuel consumption of a
large coal fired power plant, it is more than adequate for district heating
applications. The other major benefit of using biomass for small distributed
heating supplies is the reduction in sulfur emissions. Since it is seldom
cost effective to install scrubbers on small boilers, and many were built
before scrubbers were required, any small coal or oil fueled boiler will
emit significant quantities of sulfur dioxide. Since biomass has virtually
no sulfur content, replacing oil and coal in these boilers with biomass will
eliminate that source of air pollution.
Biomass also produces electricity indirectly, as methane from landfills and
digesters is burned in engines. This pathway generates about 90 TWhr/yr of
electricity. As increasingly stringent environmental regulations impose
tighter controls on the release of gasses from landfills and digesters, we
can expect a rapid growth in using this gas for power generation.
The estimate for the quantity of fossil fuels which can be replaced with
biomass is very much dependent on the assumptions made regarding how it will
be used. Because biomass is very much a democratic fuel, available to
everyone and everywhere, it is most appropriate to make no assumptions about
how it will be used. The only assumption that it is safe to make is that
where it is available someone will figure out a way to use it. On this
basis, the potential for biomass is to displace all other fuels which it can
displace economically and conveniently. For small consumers this is any fuel
which costs more than $11/mmBTU, and for larger users it will be dictated by
availability and transportation costs.
Solar
Solar electric is currently one of the more expensive methods to produce
electricity, but, to the surprise of many, it can be cost effective in many
cases. The best example is southern California, where peak electric rates
can be as high as $0.25 / kwh and various incentives are available to pay
for nearly half the cost of a PV system. Since the PV system will offset the
purchase of on-peak power, it can actually be economically attractive for a
homeowner to install a small PV system for peak-shaving. It is never cost
effective for large scale wholesale electric generation and probably will
never be. The use of solar thermal power plants could somewhat reduce the
cost of utility scale generation compared to PV, but not nearly enough to be
cost effective compared to natural gas fueled combined cycle plants. The
utilities installing large solar generating capacity are doing so only for
bragging rights, and ultimately higher electric prices will result. Nor is
PV currently cost effective in most other areas of the country where there
is less available sunshine and much lower electric prices. Despite the
economic limitations, solar PV is growing at an astounding rate.
Aside from the cost, the potential limitations for solar generated
electricity are the availability of sunshine and manufacturing capability of
equipment. Obviously sunshine is not available at night and is limited
during the winter, but neither is there any shortage of electric generating
capacity at night or during the winter. There is no point in trying to store
solar power for off-peak use, so I will consider solar power only as a
peak-shaving supply. It is the worldwide manufacturing capability which is
currently the limiting factor for solar PV. There is currently enough
capacity to manufacture approximately 2,200 MW of PV panels per year, of
which about 330 MW goes to the U.S. and much of the remainder is going to
places such as Germany which have inordinately high incentives for PV
installations.
Curiously, these ridiculous incentives are not helping either the
environment, the solar PV industry, nor the German economy. The exorbitant
incentives harm the industry by encouraging the perception that solar PV
cannot compete with other forms of generation. They harm the environment
because Germany is a poor location for solar energy, so a panel installed
there will generate less electricity than one installed in a sunnier
location. Germany also has sufficient and modern electrical generation and
much of it is connected to district heating systems leading to clean and
efficient utilization of the fuel they burn.
Many locations throughout the world rely on inefficient, outdated, and
inadequate generation sources, and would benefit much more both in terms of
electricity generated and reduced emissions from the use of those PV panels
than does Germany. In the U.S., a similar situation exists between northern
states and southern California. It is southern California which has a high
availability of sunshine combined with power shortages, high electric rates,
and problems with air pollution. Therefore any solar panel installed
somewhere else in the country is not being used to its full potential. It is
on this basis that I will evaluate the potential of solar PV power.
California's highest ever peak summer electrical demand was 52,863 MW, and
they have an average demand of approximately 30,000 MW. Solar PV is ideally
suited to supply that 23,000 MW difference between the peak and average
demand. If the total U.S. share of PV panels were installed in California,
it would take approximately 70 years before California’s peak differential
were satisfied. Regardless of how quickly solar PV grows, it will not reach
any constraint nor provide significant generation in the U.S. while
Germany’s exorbitant incentives remain in place. However, when Germany
reduces or eliminates its exorbitant incentives (as eventually it must) then
possibly as much as 1,000 MW of panels could be installed annually in the
U.S. At that rate the peak differential in California could be satisfied in
20 years.
Solar PV to supply the mobile power market will enjoy much more favorable
economic factors, but is still limited by supply. Electric cars (including
plug-in hybrids) are rapidly growing in popularity and PV can easily be
incorporated to extend their range and provide recharging flexibility. The
kind of people who would buy an electric car are very likely to find on
board PV panels desirable and worth a premium in cost. Compared to a
gasoline engine which produces power at approximately $0.30/kw-hr, PV panels
generating power at $0.20/kw-hr are a good deal. Judging by sales of hybrid
cars in the US, it does not seem unreasonable to expect that 10,000
cars/year could be sold with 2kW each of solar panels. This would reduce
gasoline consumption by 1.8 million gallons/year/year.
Ocean / River Current
Conventional hydropower has long been used to supply electricity for
stationary use, but it is expected to diminish in coming years because of
environmental concerns. Free-flowing hydropower, which doesn't required any
dam, may somewhat replace it. While actual installations of free-flowing
hydroelectric production are limited to a handful of demonstration projects,
it could be, to the east coast, what wind power is to the Midwest. The
limitations to free-flowing hydropower will not be physical or technical,
they will be political. At this time it is difficult to predict what the
major impediments will be to development of free-flowing hydropower, but it
is likely to be the same factors which inhibit off-shore wind.
These are such concerns as visual impact, environmental disturbance, damage
to marine life, and competing uses for the area. These are not actually
limitations of the energy source per se, and they are constantly changing,
so I will not try to evaluate them. From a technical standpoint, the
development will be limited by availability of equipment. Currently there
are no commercial offerings for free-flowing hydropower generators. If a
major OEM undertook a development program now, they could probably have a
prototype 100 MW barge mounted hydrogenerator ready in 10 years, with a
commercial product 5 years later. We could then expect at least 5-10 years
of limited use before the concept gains industry acceptance. Therefore,
free-flowing hydropower will probably make no significant contribution to
our energy production in the next 30 years.
The result of all this is that renewable energy will be able to displace
varying amounts of conventional fuels, and that the amount depends primarily
on the end use of the fuel. The table below shows my prediction for the
fraction of energy which renewables can contribute to each category in the
foreseeable future:
As can be seen the most painful limitation is with transportation fuels.
These are the most expensive and the most difficult to substitute. It is
therefore highly desirable that any liquid fuel which is currently used in a
stationary application be immediately replaced with a solid or gaseous fuel.
This also shows that using electricity to power transportation via electric
cars, plug-in hybrids, or electric trains and busses will not only decrease
petroleum consumption, it will also increase the fraction of renewable
energy used for transportation. The important principal to learn from this
study is that not all energy (including renewable forms) is created equal.
In order to maximize the fraction of renewable energy which can be put to
use it is imperative that each form of energy be used in it's most effective
manner.
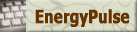
Copyright © 2002-2006,
CyberTech, Inc. - All rights reserved.
|